How Airport Distance Calculations Really Work A Look at Great Circle Routes and Flight Planning
How Airport Distance Calculations Really Work A Look at Great Circle Routes and Flight Planning - Great Circle Math Behind JFK to LHR Flight Distance 4123 Nautical Miles
The shortest distance between JFK and LHR, as determined by great circle math, is roughly 3,452 miles (or 5,555 kilometers) which translates to about 2,999 nautical miles. This optimal path, which leans northeast at about a 51-degree angle, is a direct result of the Earth's spherical nature. The calculation relies on both airports' latitude and longitude, utilizing complex formulas based on spherical trigonometry. In the realm of aviation, understanding these great circle routes is crucial. They are the foundation of efficient flight planning, offering the shortest possible routes which reduce flight time and fuel consumption. Specialized tools like the Great Circle Mapper are employed to easily calculate these distances, showcasing their central role in the precision required in modern air travel.
1. The 4,123 nautical mile distance from JFK to LHR is derived from the great circle formula, a method that treats the Earth as a sphere, offering a more realistic depiction of flight paths compared to simple map-based lines. It's interesting to note that this spherical approach is crucial for accurate distance calculations across long distances.
2. A degree of latitude translates to about 60 nautical miles regardless of location, whereas a degree of longitude is variable depending on the latitude. This variation is a critical aspect of finding the most efficient routes, highlighting a fascinating interaction between the Earth's geometry and flight planning.
3. The JFK-LHR great circle route crosses multiple time zones, introducing complexity to flight scheduling. Arrival times become more challenging to manage, and operational coordination must consider this time difference, a good reminder of the global implications of even seemingly simple flights.
4. The Earth isn't a perfect sphere—its shape is closer to an oblate spheroid, slightly flattened at the poles and bulging at the equator. This means the great circle calculation, while useful, needs to be aware of this nuance. It's a good reminder that assumptions about Earth's shape, while helpful in simple calculations, need to be revised for extremely accurate calculations.
5. GPS relies on satellites to calculate precise positions and distances. For flights like JFK-LHR, the calculations must constantly adjust for variables like altitude and atmospheric conditions, offering a fascinating illustration of the complexities inherent in precise position determination.
6. The theoretical great circle route isn't necessarily the actual path a plane follows. Factors such as weather, air traffic control, and even political considerations can lead airlines to choose routes that are longer than the optimal great circle distance. This is an example of the conflict between ideal theoretical routes and practical, real-world considerations.
7. Wind conditions, such as the Jet Stream, are also relevant. To take advantage of tailwinds or mitigate headwinds, flights can alter altitude or route, impacting total distance and flight time. It demonstrates the intricate dance between air currents and optimal flight planning.
8. While the great circle route offers the shortest distance, the actual routes are often longer due to controlled airspace and navigational restrictions. These factors can sometimes increase the flight distance by around 10%, reinforcing the concept that practical flight planning incorporates multiple competing constraints.
9. The ICAO has laid out procedures for flight planning, considering navigational aids and airspace classification. This framework shows how theoretical mathematical concepts are integrated with established operational guidelines in real-world flight paths.
10. Advances in navigation technology like RNP enable aircraft to fly more precise paths that may not perfectly match the theoretical great circle route. This precision is especially useful in crowded airspaces, again showing the evolution of flight planning beyond simple mathematical models.
How Airport Distance Calculations Really Work A Look at Great Circle Routes and Flight Planning - Why Aircraft Follow Curved Lines on Flat Maps During Pacific Routes
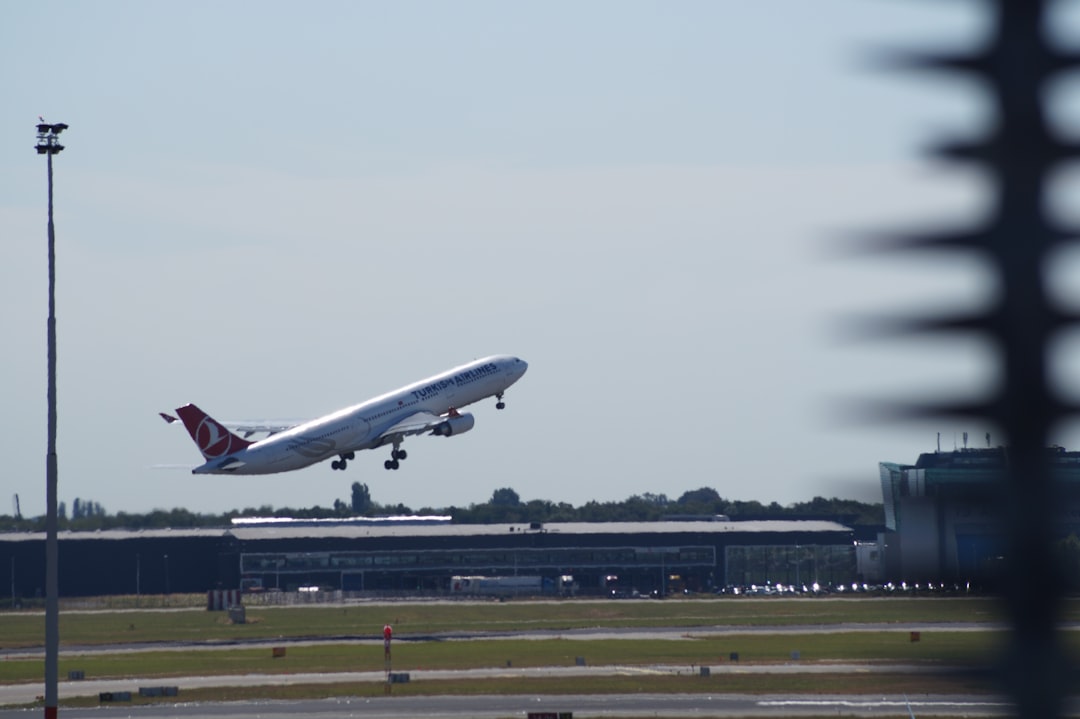
When viewing Pacific flight routes on a standard flat map, you'll notice aircraft paths often appear curved. This curvature is a direct result of the Earth's spherical shape. The shortest distance between two points on a sphere isn't a straight line but rather a curved arc, known as a great circle route. These routes are crucial for flight planning because they minimize flight time and fuel consumption, making them highly desirable for long-distance flights.
However, while great circle routes are the most efficient on a globe, they appear curved on a flat map simply due to the challenges of representing a 3D object on a 2D surface. This distortion can be a bit confusing to the casual observer.
Furthermore, other factors often influence the actual flight paths taken by aircraft. These include the presence of jet streams which can provide a speed boost, air traffic control regulations, and even political considerations that can mandate diversions around certain areas. While the idealized great circle route provides a starting point for flight planning, the actual route can deviate due to these practical elements.
In essence, even though curved lines might seem counterintuitive, they reflect a strategic approach to maximizing flight efficiency across vast distances like those encountered over the Pacific Ocean.
When looking at a flat map of Pacific routes, it's common to see aircraft paths depicted as curved lines. This is a direct result of the Earth's spherical shape and the way navigation systems try to follow the shortest distance—great circle routes. These routes appear as straight lines on a globe but get distorted when flattened onto a map, producing a visually curved path.
The shortest distance between two points on a sphere is called a great circle, and this path often doesn't translate to a straight line on a flat map. This is due to the challenges of projecting a 3D shape onto a 2D surface. Since the distance between degrees of longitude changes with latitude, this distortion becomes more pronounced at higher latitudes. It's a clear example of how map projections can significantly impact the way we understand distances and routes.
While a flight plan may seem straightforward on a navigation chart, it's actually composed of various waypoints that are carefully chosen to comply with safety regulations and traffic control procedures. This emphasizes the tension between theoretically efficient paths and the practicalities of keeping flights safe and compliant.
Flights across the Pacific often involve adjusting the aircraft's altitude to take advantage of prevailing winds, such as the Jet Stream. This further complicates the flight path, resulting in more curves on the map as the plane navigates for optimal wind conditions. This optimization involves a constant balancing act between minimizing fuel consumption and adhering to great circle principles.
Organizations like the International Air Transport Association (IATA) have established detailed procedures for aircraft flight planning, which consider the complexities of variable time zones and weather conditions. These detailed procedures help ensure efficient, safe flight operations and demonstrate a fascinating mix of technical knowledge and operational experience in creating routes.
Flight crews and dispatchers scrutinize onboard data, including fuel consumption, to ensure flights stay economical while still adhering to the general principles of great circle routes. This underscores that while the optimal route theoretically minimizes distance, actual flights need to consider practical factors such as economic feasibility and operational efficiency.
The density of air traffic can have a large impact on route deviations, causing aircraft to steer around congested areas. These diversions often make the routes look even more curved on flat maps. It's a great illustration of how theoretical models and real-world constraints influence actual flight paths.
While great circle routes provide the shortest theoretical distances, the actual time taken by a flight can vary quite a bit based on several factors. These include changes in airspeed and the required climbs or descents during the flight. It's a reminder that, in reality, a flight's efficiency is about much more than just the shortest distance.
Improvements in flight planning automation and artificial intelligence are now enabling airlines to dynamically adapt flight routes in real-time. This optimization capability, while leading to more efficient routes overall, still results in paths that often appear curved on flat maps. This highlights a continuous effort to refine flight planning techniques and balance mathematical optimality with operational constraints.
How Airport Distance Calculations Really Work A Look at Great Circle Routes and Flight Planning - Aircraft Fuel Calculations Based on Great Circle Distance Examples
Aircraft fuel calculations often rely on great circle distances to estimate fuel burn for flights. Finding the shortest path between two points on Earth's surface, the great circle route, is crucial for accurate fuel estimations. The calculations usually involve the Haversine formula, along with adjustments for aircraft type, weight, and even the nature of the flight itself. These fuel estimates often take the form of a quadratic equation, adjusting for how fuel consumption changes as flight distance varies. It's not just about fuel—these calculations also help project the carbon footprint of flights. To estimate CO2 emissions, the fuel calculations incorporate information about cargo loads and passenger numbers (load factors), which are critical aspects of ICAO traffic data. The overall aim of these fuel calculation methods is twofold: they're critical for cost efficiency in a field where fuel costs are substantial, and they also promote more sustainable flying, as every ounce of fuel saved helps reduce emissions and improves fuel efficiency. While some methods involve standardizing aircraft types and using linear interpolation for certain distances, the core idea remains consistent—use the great circle distance and relevant factors to accurately predict fuel needs.
1. Great circle distances are fundamental to aircraft fuel calculations, but the process isn't as straightforward as simply measuring the shortest distance. Aircraft altitude and speed also play a role, as optimizing these factors can lead to more efficient fuel consumption, especially on longer flights. This indicates that a nuanced approach, beyond the simple distance calculation, is required.
2. While great circle routes represent the theoretically optimal path, factors like atmospheric resistance, changes in altitude during flight, and the aircraft's weight can lead to deviations from the calculated path. This variation in actual flight path adds a layer of complexity to fuel calculations that needs to be addressed. It seems the simple theory of great circle math needs to be carefully adapted to reflect actual flight conditions.
3. The impact of air density on fuel consumption can be surprising. As aircraft ascend, the air becomes less dense, meaning engines can operate more efficiently. However, this also requires careful fuel management to ensure optimal flight performance. It's clear that there are unique challenges to fuel optimization at different altitudes and in various weather conditions.
4. Great circle routes, though mathematically optimal, don't always align with the most fuel-efficient route due to the unpredictable nature of wind patterns. The Jet Stream, for instance, can significantly influence flight planning decisions, potentially requiring alterations to routes, and therefore, fuel strategies. This shows that fuel consumption needs to adapt to dynamic conditions rather than simple mathematical results.
5. Interestingly, international aviation regulations often mandate fuel reserves in flight planning, typically 5-10% above the estimated requirement for a great circle route. This practice adds a layer of complexity that extends beyond the basic principles of great circle math. It is concerning that international standards sometimes override calculations that minimize fuel consumption and seem to prefer an overly cautious approach.
6. Fuel efficiency tends to improve on longer flights, leading to a peculiar outcome where short-haul flights might paradoxically consume more fuel per distance travelled compared to longer journeys. This potentially challenges the applicability of focusing solely on great circle distance when planning short flights. It brings into question whether the optimization focus of great circle math is always beneficial.
7. When air traffic control directives mandate a deviation from the great circle path, additional considerations emerge in fuel calculations. Increased distance or changes in altitude required to meet these demands can impact the original fuel estimates based on great circle distance. It's evident that air traffic control, a critical factor for safety, can conflict with the principles of fuel efficiency.
8. The concept of reserve fuel, required to handle unexpected situations, further complicates fuel calculations. It forces flight planners to incorporate great circle distance considerations within a broader context of safety protocols. It also highlights the risks of simply trusting mathematically derived fuel consumption estimates.
9. Aircraft performance, influenced by its weight and engine specifications, is crucial for fine-tuning fuel estimations derived from great circle distances. This emphasizes the need for tailored assessments rather than relying on generalized models. It shows that fuel calculation isn't a 'one size fits all' situation, requiring aircraft-specific information.
10. Environmental factors like temperature and humidity can impact engine performance and therefore fuel consumption. Modern aircraft use real-time data to adjust fuel use based on weather conditions, striving to stay aligned with the flight plan established through great circle distances, but doing so with an understanding of real world conditions. This shows that ongoing, dynamic optimization is needed to combine theoretical ideals and practical concerns.
How Airport Distance Calculations Really Work A Look at Great Circle Routes and Flight Planning - Impact of Earth Curvature on North Atlantic Track System Routes
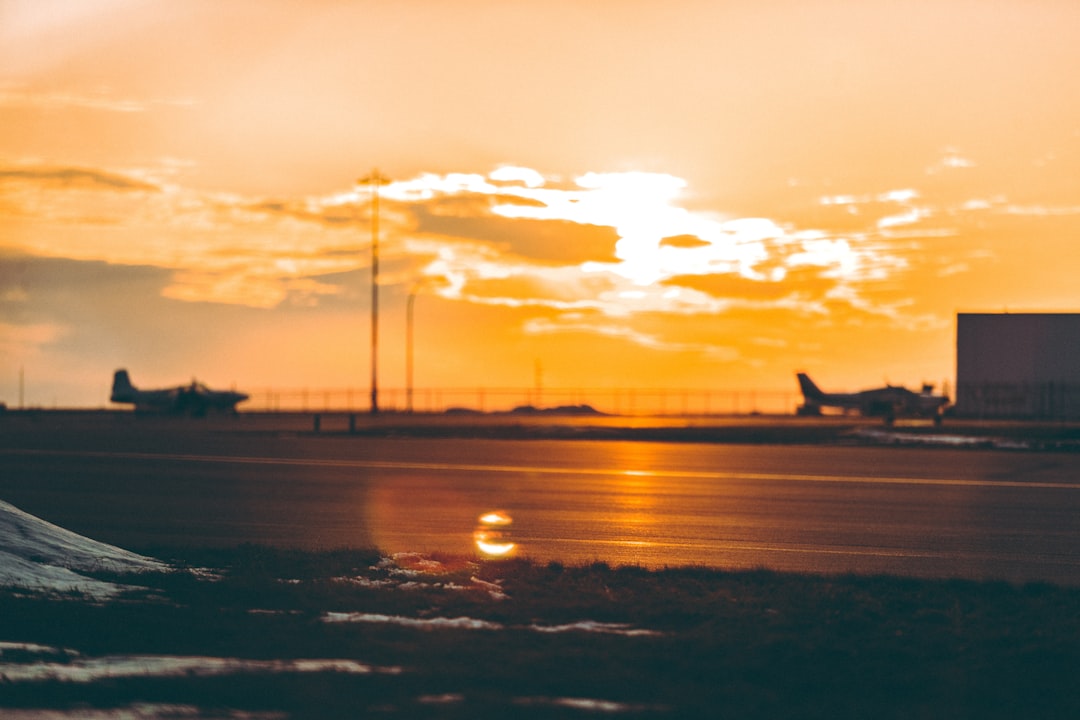
The North Atlantic Track System (NATS) provides a clear illustration of how Earth's curvature significantly influences flight planning. The system relies heavily on the concept of great circle routes, aiming to minimize flight distance and fuel burn. However, these ideal, mathematically-derived routes are often adjusted in practice. The need for this adaptation stems from the ever-changing jet streams and atmospheric conditions that dominate the North Atlantic. These factors not only cause the flight paths to appear curved on standard maps, but also lead to dynamic decision-making by both pilots and air traffic controllers. Additionally, weather patterns associated with phenomena like the North Atlantic Oscillation further complicate these decisions, influencing fuel usage and resulting emissions. This emphasizes the intricate balance between theoretical efficiency and real-world constraints when designing and executing flights across the North Atlantic. Although the great circle route offers the foundation for planning, safety and efficiency considerations demand adjustments and adaptability, demonstrating that the practical aspects of air travel often override the most mathematically ideal routes.
1. The Earth's curvature significantly impacts transatlantic flights, particularly when considering the need to avoid restricted airspace. This can result in actual flight paths that deviate considerably from the mathematically ideal great circle routes, illustrating a crucial divergence between theory and practice.
2. The North Atlantic Track System (NATs) is a prime example of how air traffic management accounts for Earth's curvature on a large scale. While the concept of a great circle route aims for optimal distance, the NAT system introduces specific routes that prioritize both safety and efficiency within this broader context.
3. The Earth's curvature introduces a phenomenon called "circular error probable" (CEP) in aviation, a statistical measure of flight path accuracy. This emphasizes the importance of considering the Earth's shape for ensuring precise navigation.
4. When navigating curved routes across the North Atlantic, pilots use "true heading" in contrast to "magnetic heading." This practice highlights the continuous adjustments needed to account for both the Earth's curvature and magnetic variations. This reveals that flight path navigation involves a fascinating interplay of several variables.
5. Earth's curvature not only affects distance but also alters the visual landscape for pilots. The curved horizon means that pilots must rely more on instruments, like GPS, rather than visual cues when flying over vast stretches of ocean. This underscores the crucial role of modern navigational aids for safe transatlantic flight.
6. Communication protocols for air traffic control over the North Atlantic are tailored to account for the Earth's curvature. This leads to specific operational practices that help maintain safe distances between aircraft. It's interesting to note that while a flat map might suggest otherwise, the curved nature of the Earth requires special attention to maintain safe separation.
7. When designing North Atlantic flight routes, the curvature of the Earth is factored in by optimizing aircraft altitudes. Flying at higher altitudes can reduce atmospheric drag which is influenced by Earth's curvature. This shows that optimizing routes takes into account a complex interaction between atmospheric factors and the shape of the Earth.
8. The impact of the Earth's curvature is also evident in fuel load calculations, especially for longer flights across the North Atlantic. Airlines must adjust their models to account for the increased fuel requirements associated with these longer, curved paths, revealing that even seemingly simple concepts like fuel calculation are altered by the Earth's shape.
9. By understanding the Earth's curvature, it becomes clear why some flight paths appear longer on traditional maps than they might in a simple theoretical framework. This difference matters for accurate operational planning and managing passenger expectations regarding flight durations, showing how a spherical Earth complicates flight logistics.
10. Due to the impact of Earth's curvature, pilots and flight planners must adopt an adaptive approach to navigation. This involves frequently recalibrating routes to respond to the specific effects of the Earth's curvature in different segments of a North Atlantic flight. It’s a demonstration of how the successful operation of flight relies on a fascinating mixture of scientific understanding and practical know-how.
How Airport Distance Calculations Really Work A Look at Great Circle Routes and Flight Planning - Waypoint Planning Through High Latitude Routes Above 60 Degrees
Navigating through high-latitude routes above 60 degrees introduces a unique set of considerations for waypoint planning in aviation. The Earth's curvature plays a more significant role in these regions, affecting how aircraft paths are plotted and flown. While great circle routes offer the shortest theoretical distance, practical flight planning in high latitudes often requires deviations from these ideal paths due to factors like rapidly shifting weather and the unique demands of polar air traffic management. The constantly changing atmospheric conditions and potential for extreme weather events further complicate navigation, necessitating precise and frequent adjustments to flight paths as planes move through these regions. Therefore, successful waypoint planning in these areas requires a delicate balance between adhering to the mathematically optimal paths and remaining mindful of the practical challenges and operational constraints specific to high-latitude flight. This balance ensures safety and efficiency amidst a complex set of navigational factors.
When planning flights over high latitude routes, above 60 degrees, we encounter a unique set of challenges due to the Earth's geometry. The convergence of lines of longitude near the poles can create a situation where the shortest distance between two points, as calculated by the usual great circle methods, might not be the most accurate or practical. This highlights a potential limitation of simple great circle calculations in these high latitude areas, especially if they don't specifically account for the changing distance relationship between degrees of longitude.
In these remote areas, reliance on technology becomes crucial for maintaining safe and accurate flights. Navigational aids on the ground are scarce or non-existent, making satellite navigation, particularly GPS, critically important. This also means consistent, reliable GPS coverage across the polar regions is paramount.
Flying in high latitude regions introduces fascinating challenges. Polar events, such as the Aurora Borealis or Aurora Australis, can have a disruptive effect on radio communications, which is problematic for air traffic control and pilot navigation. Maintaining communication and navigating safely becomes a continuous process of adapting to the unique and often unpredictable environment.
The Earth's tilt and the seasonal changes it generates play a huge role in route planning above 60 degrees. Shifts in jet streams and variations in weather can be dramatic, making route optimization a dynamic process. Airlines must take these variations into account when planning and adjust flights based on the season rather than solely relying on a static great circle approach.
The long periods of daylight or darkness at high latitudes create another challenging aspect. The changing light cycles not only affect visual navigation but also have a profound impact on flight crew schedules. This requires meticulous planning and coordination to ensure adequate crew rest and safety.
High-latitude flight planning intersects with multiple countries’ airspaces, leading to an even more complex regulatory environment. The procedures for each country can be quite different, demanding careful coordination and awareness to remain compliant.
Temperature fluctuations are extreme in these areas and impact aircraft performance and fuel efficiency. Colder air at higher altitudes does increase lift, but managing fuel consumption efficiently at those altitudes is more difficult and requires more complex calculations. This unique aspect of flight requires us to adapt and refine standard fuel calculation approaches.
As you move toward the poles, the relationship between true north and magnetic north becomes increasingly important. Instruments need to be adjusted accordingly, adding another layer of complexity to waypoint management and navigation.
The presence of strong winds, particularly the polar jet stream, creates an opportunity to improve fuel efficiency. Airlines can dynamically adjust flight paths to capitalize on favorable winds and potentially save a significant amount of fuel.
Sophisticated flight planning software has become indispensable for high-latitude routes. The complex interplay of fuel efficiency, changing wind patterns, and regulatory requirements necessitates a powerful toolset that can generate routes that remain optimally safe and efficient, taking into account the unique constraints of these challenging environments.
It's clear that continued research and development are needed in the field of aviation to refine our understanding of these dynamic environments and to adapt navigation and flight planning procedures for increasingly efficient and safe operations in high-latitude airspace.
How Airport Distance Calculations Really Work A Look at Great Circle Routes and Flight Planning - Weather Pattern Effects on Great Circle Route Modifications
Weather patterns play a significant role in how Great Circle routes are actually flown. Flight planning must account for things like jet streams, which can either help or hinder a plane's progress. When weather conditions are dynamic, especially in areas prone to strong storms or turbulence, pilots might need to adjust their flight path in real-time to make sure the flight is safe and uses fuel efficiently. While Great Circle routes are designed to be the shortest distance, real-world weather can force planes onto more complicated paths that don't perfectly match the initial mathematical ideal. This means understanding how weather impacts flight planning is extremely important for operating aircraft in a safe and effective way. The idea of the shortest route is constantly being challenged by the realities of flight, forcing a re-evaluation of initial flight plans to best match the conditions of the flight.
1. Weather conditions can significantly alter a plane's actual flight path, sometimes adding hundreds of miles to the journey. These changes are especially pronounced when dealing with unpredictable wind patterns and unexpected storm systems. Airlines are limited in how far ahead they can accurately predict these types of events.
2. The Jet Stream, a high-altitude wind current moving generally from west to east, can significantly impact fuel efficiency. Aligning a flight path to take advantage of tailwinds from the Jet Stream can reduce fuel consumption by as much as 10%. This illustrates the important role weather can play in flight planning and optimization.
3. When flying through areas with severe weather turbulence, flight paths need to be adjusted in real-time to avoid unsafe conditions. This can cause the planned great circle route to shift drastically, highlighting the need for flexible flight paths to prioritize safety and passenger comfort.
4. The North Atlantic is known for its unique weather systems, shaped by the ocean currents that create turbulent and variable weather conditions. Pilots are often forced to significantly deviate from ideal great circle routes due to these dynamic and unpredictable weather patterns. It's a strong reminder that aircraft flight planning needs to incorporate adaptive strategies.
5. Fluctuations in atmospheric pressure can influence the accuracy of altitude adjustments, which in turn affects fuel consumption. Engineers constantly refine their models to account for these atmospheric complexities and achieve optimal flight performance. Understanding these factors is crucial for accurate flight planning.
6. The curvature of the Earth itself introduces unique challenges when planning flights across diverse weather zones. Aircraft traveling from tropical to polar regions not only need to consider distance, but also the dramatic shifts in weather patterns across these latitudinal changes. The impact of these weather variations needs to be factored into great circle routes.
7. Pilots rely on real-time satellite data to monitor and forecast changing weather during flights. This allows them to maintain safe and efficient flight paths, highlighting the importance of advanced technologies beyond the simpler great circle calculations.
8. Atmospheric instability, particularly where warm and cold fronts converge, can cause sudden and unpredictable turbulence, requiring quick route adjustments. This is a significant challenge that adds a layer of complexity to great circle route planning, demonstrating the importance of integrating meteorological understanding into route optimization.
9. When planning high-altitude flights, factors like temperature variations at different altitudes can affect engine efficiency. Aircraft can experience decreased performance in warmer air at high altitudes, meaning the optimal great circle path might not always be the most operationally efficient due to unexpected temperature variations.
10. Predictive analytics are becoming increasingly sophisticated, allowing for more accurate weather forecasting and improved pre-flight planning. This proactive approach strives to combine traditional great circle calculations with real-world weather data, offering a more refined solution to the complexities of aviation navigation.
More Posts from :